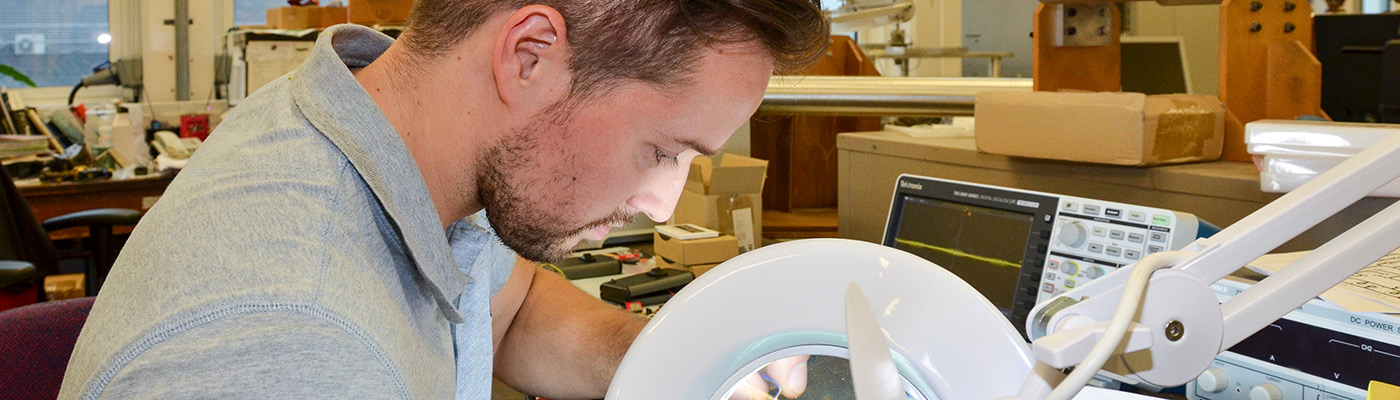
Research themes
Research within the Department of Physics and Astronomy is separated into four key themes.
With access to an incredible array of facilities - including Jodrell Bank Observatory - and strong links to centres and institutes around the world, we are able to research the full breadth of physics.
Discover our themes in greater detail, and find out which supervisors specialise in your area of interest.